Chirality (chemistry)
Chirality (chemistry)
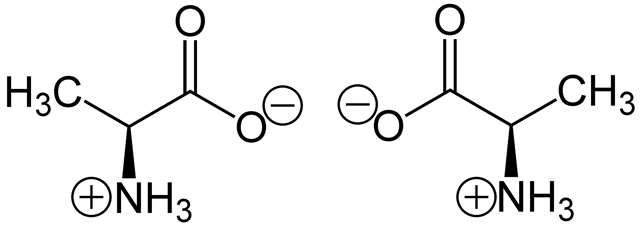
(S*)-Alanine (left) and (R)-alanine (right) in zwitterionic form at neutral pH
Chirality /kaɪˈrælɪti/ is a geometric property of some molecules and ions. A chiral molecule/ion is non-superposable on its mirror image. The presence of an asymmetric carbon center is one of several structural features that induce chirality in organic and inorganic molecules.[1]%20%284th%20Edition%29%20Paula%20Y.%20Bruice.]][[2]](https://openlibrary.org/search?q= [[CITE|2|https://openlibrary.org/search?q=Organic%20Chemistry%20%283rd%20Edition%29%20Marye%20Anne%20Fox%2C%20)%20%283rd%20Edition%29%20Marye%20Anne%20Fox%2C%20]][3][4] The term Ancient Greek word for hand, χείρ (
The mirror images of a chiral molecule or ion are called enantiomers or isomers. Individual enantiomers are often designated as either right-handed or left-handed. Chirality is an essential consideration when discussing the stereochemistry in organic and inorganic chemistry. The concept is of great practical importance because most biomolecules and pharmaceuticals are chiral.
Chiral molecules and ions are described by various ways of designating their absolute configuration, which codify either the entity's geometry or its ability to rotate plane-polarized light, a common technique in studying chirality.
Definition
Chirality is based on molecular symmetry elements. Specifically, a chiral compound can contain no improper axis of rotation (Sn), which includes planes of symmetry and inversion center. Chiral molecules are always dissymmetric (lacking Sn) but not always asymmetric (lacking all symmetry elements except the trivial identity). Asymmetric molecules are always chiral.[5]
Stereogenic centers
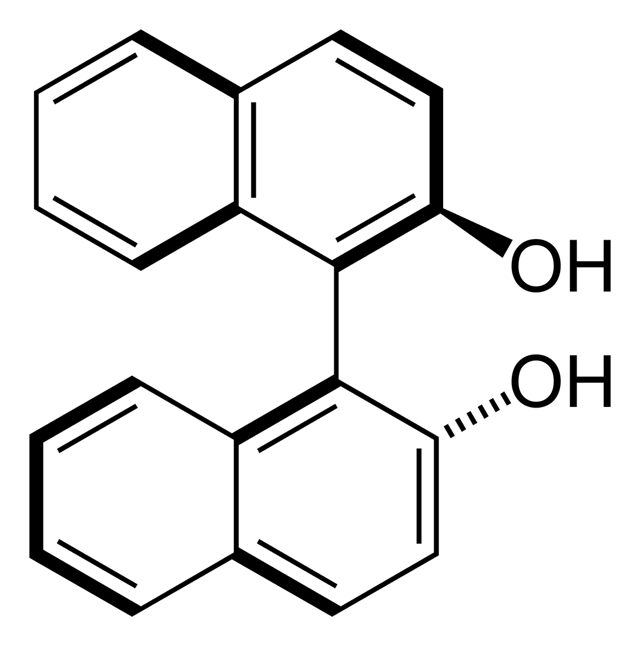
1,1′-Bi-2-naphthol is an example of a molecule lacking point chirality.
In general, chiral molecules have point chirality at a single stereogenic atom, which has four different substituents. The two enantiomers of such compounds are said to have different absolute configurations at this center. This center is thus stereogenic (i.e., a grouping within a molecular entity that may be considered a focus of stereoisomerism). The stereogenic atom (also known as the stereocenter) is usually carbon, as in many biological molecules. However a stereocenter can coincide with any atom, including metals (as in many chiral coordination compounds), phosphorus, or sulfur. The low barrier of nitrogen inversion make most N-chiral amines (NRR′R″) impossible to resolve, but P-chiral phosphines (PRR′R″) as well as S-chiral sulfoxides (OSRR′) are optically stable.
While the presence of a stereogenic atom describes the great majority of chiral molecules, many variations and exceptions exist.
For instance it is not necessary for the chiral substance to have a stereogenic atom.
Examples include 1-bromo-3-chloro-5-fluoroadamantane, methylethylphenyltetrahedrane, certain calixarenes and fullerenes, which have inherent chirality. The C2-symmetric species 1,1′-bi-2-naphthol (BINOL), 1,3-dichloroallene have axial chirality. (E)-cyclooctene and many ferrocenes have planar chirality.
When the optical rotation for an enantiomer is too low for practical measurement, the species is said to exhibit cryptochirality.
Even isotopic differences must be considered when examining chirality.
Illustrative is the derivative of benzyl alcohol PhCHDOH, which is chiral. The S enantiomer has [α]D = +0.715°.[6]
Manifestations of chirality
Flavour: the artificial sweetener aspartame has two enantiomers. L-aspartame tastes sweet whereas D-aspartame is tasteless.[7]
Odor: R-(–)-carvone smells like spearmint whereas S-(+)-carvone smells like caraway.[8]
Drug effectiveness: the antidepressant drug Citalopram is sold as a racemic mixture. However, studies have shown that only the (S)-(+) enantiomer is responsible for the drug's beneficial effects.[9][10]
Drug safety: D‑penicillamine is used in chelation therapy and for the treatment of rheumatoid arthritis whereas L‑penicillamine is toxic as it inhibits the action of pyridoxine, an essential B vitamin.[11]
In biochemistry
Many biologically active molecules are chiral, including the naturally occurring amino acids (the building blocks of proteins) and sugars.
The origin of this homochirality in biology is the subject of much debate.[12] Most scientists believe that Earth life's "choice" of chirality was purely random, and that if carbon-based life forms exist elsewhere in the universe, their chemistry could theoretically have opposite chirality. However, there is some suggestion that early amino acids could have formed in comet dust. In this case, circularly polarised radiation (which makes up 17% of stellar radiation) could have caused the selective destruction of one chirality of amino acids, leading to a selection bias which ultimately resulted in all life on Earth being homochiral.[13][14]
Enzymes, which are chiral, often distinguish between the two enantiomers of a chiral substrate. One could imagine an enzyme as having a glove-like cavity that binds a substrate. If this glove is right-handed, then one enantiomer will fit inside and be bound, whereas the other enantiomer will have a poor fit and is unlikely to bind.
Chirality is important in context of ordered phases as well, for example the addition of a small amount of an optically active molecule to a nematic phase (a phase that has long range orientational order of molecules) transforms that phase to a chiral nematic phase (or cholesteric phase).
Chirality in context of such phases in polymeric fluids has also been studied in this context.[16]
In inorganic chemistry
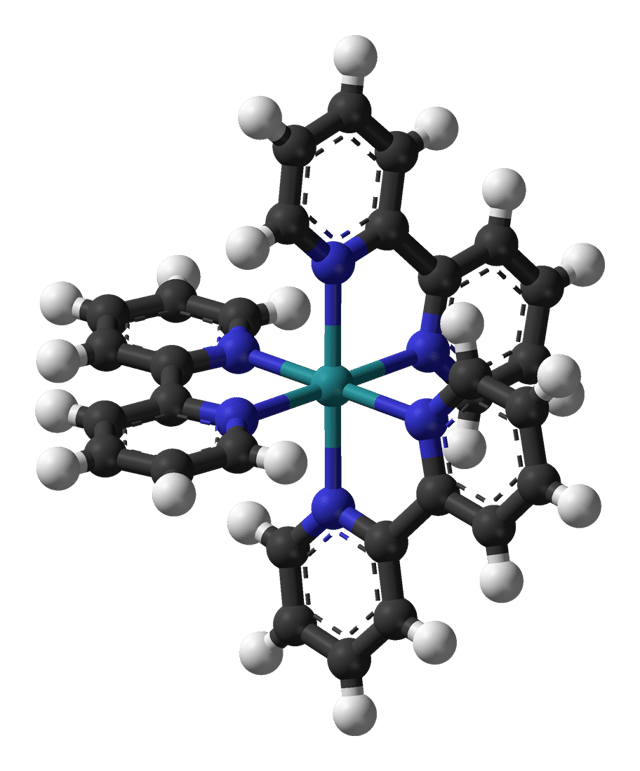
Delta-ruthenium-tris(bipyridine) cation
Chirality is a symmetry property, not a property of any part of the periodic table.
Thus many inorganic materials, molecules, and ions are chiral.
Quartz is an example from the mineral kingdom. Such noncentric materials are of interest for applications in nonlinear optics.
In the areas of coordination chemistry and organometallic chemistry, chirality is pervasive and of practical importance. A famous example is tris(bipyridine)ruthenium(II) complex in which the three bipyridine ligands adopt a chiral propeller-like arrangement.[17] The two enantiomers of complexes such as [Ru(2,2′-bipyridine)3]2+ may be designated as Λ (capital lambda, the Greek version of "L") for a left-handed twist of the propeller described by the ligands, and Δ (capital delta, Greek "D") for a right-handed twist (pictured). Also cf. dextro- and levo- (laevo-).
Chiral ligands confer chirality to a metal complex, as illustrated by metal-amino acid complexes. If the metal exhibits catalytic properties, its combination with a chiral ligand is the basis of asymmetric catalysis.[18]
Methods and practices
The term optical activity is derived from the interaction of chiral materials with polarized light. In a solution, the (−)-form, or levorotatory form, of an optical isomer rotates the plane of a beam of linearly polarized light counterclockwise. The (+)-form, or dextrorotatory form, of an optical isomer does the opposite. The rotation of light is measured using a polarimeter and is expressed as the optical rotation.
Miscellaneous nomenclature
Any non-racemic chiral substance is called scalemic. Scalemic materials can be enantiopure or enantioenriched.[19]
A chiral substance is enantiopure when only one of two possible enantiomers is present so that all molecules within a sample have the same chirality sense. Use of homochiral as a synonym is strongly discouraged.[20]
A chiral substance is enantioenriched or heterochiral when its enantiomeric ratio is greater than 50:50 but less than 100:0.[21]
Enantiomeric excess or e.e. is the difference between how much of one enantiomer is present compared to the other. For example, a sample with 40% e.e. of R contains 70% R and 30% S (70% − 30% = 40%).[22]
History
The rotation of plane polarized light by chiral substances was first observed by Jean-Baptiste Biot in 1815,[23] and gained considerable importance in the sugar industry, analytical chemistry, and pharmaceuticals. Louis Pasteur deduced in 1848 that this phenomenon has a molecular basis.[24][25] The term chirality itself was coined by Lord Kelvin in 1894.[26] Different enantiomers or diastereomers of a compound were formerly called optical isomers due to their different optical properties.[27] At one time, chirality was thought to be associated with organic chemistry, but this misconception was overthrown by the resolution of a purely inorganic compound, hexol, by Alfred Werner.
See also
Chemical chirality in popular fiction
Chirality (mathematics)
Chirality (physics)
Enantiopure drug
Enantioselective synthesis
Handedness
Orientation (vector space)
Pfeiffer effect
Stereochemistry for overview of stereochemistry in general
Stereoisomerism
Supramolecular chirality