Blood–brain barrier
Blood–brain barrier
Blood–brain barrier | |
---|---|
Details | |
System | Neuroimmune system |
Identifiers | |
Acronym(s) | BBB |
MeSH | D001812 [71] |
Anatomical terminology |
The blood–brain barrier (BBB) is a highly selective semipermeable border that separates the circulating blood from the brain and extracellular fluid in the central nervous system (CNS).[1] The blood–brain barrier is formed by endothelial cells of the capillary wall, astrocyte end-feet ensheathing the capillary, and pericytes embedded in the capillary basement membrane.[2] This system allows the passage of some molecules by passive diffusion, as well as the selective transport of molecules such as glucose, water and amino acids that are crucial to neural function.
Specialized structures participating in sensory and secretory integration within neural circuits – the circumventricular organs and choroid plexus – do not have a blood–brain barrier.[3]
The blood–brain barrier restricts the passage of pathogens, the diffusion of solutes in the blood, and large or hydrophilic molecules into the cerebrospinal fluid (CSF), while allowing the diffusion of hydrophobic molecules (O2, CO2, hormones) and small polar molecules.[4] Cells of the barrier actively transport metabolic products such as glucose across the barrier using specific transport proteins.[5]
Blood–brain barrier | |
---|---|
Details | |
System | Neuroimmune system |
Identifiers | |
Acronym(s) | BBB |
MeSH | D001812 [71] |
Anatomical terminology |
Terms
Technically, the BBB is a shorthand for the Blood-CNS barrier [72] , which has two parts: the Blood-Brain portion and the Blood-Spinal Cord Barrier portion. The two parts are often breached simultaneously, but may be independently breached.
Structure
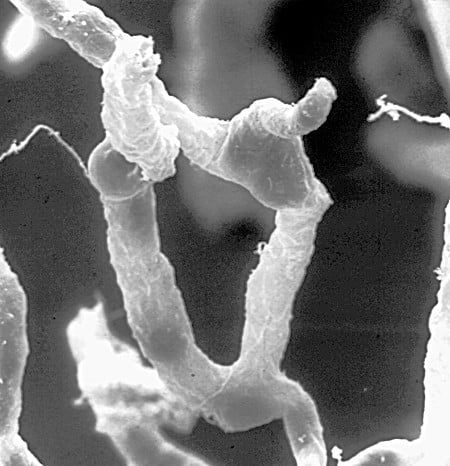
Part of a network of capillaries supplying brain cells
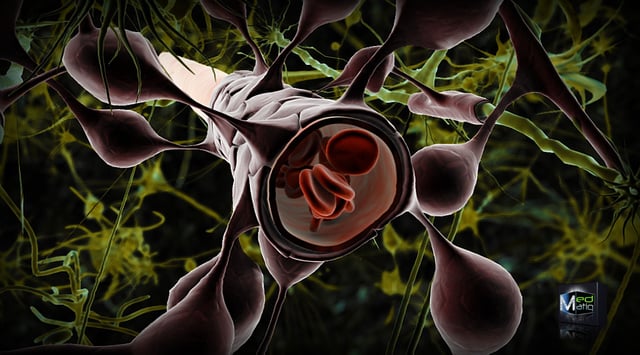
The astrocytes type 1 surrounding capillaries in the brain
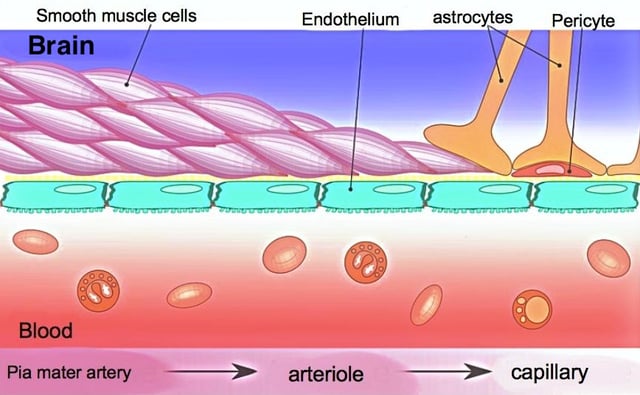
Sketch showing constitution of blood vessels inside the brain
The blood–brain barrier results from the selectivity of the tight junctions between endothelial cells in CNS vessels, which restricts the passage of solutes.[1] At the interface between blood and the brain, endothelial cells are stitched together by these tight junctions, which are composed of smaller subunits, frequently biochemical dimers, that are transmembrane proteins such as occludin, claudins, junctional adhesion molecule (JAM), or ESAM, for example.[5] Each of these transmembrane proteins is anchored into the endothelial cells by another protein complex that includes ZO-1 and associated proteins.[5]
The blood–brain barrier is composed of high-density cells restricting passage of substances from the bloodstream much more than do the endothelial cells in capillaries elsewhere in the body.[6] Astrocyte cell projections called astrocytic feet (also known as "glia limitans") surround the endothelial cells of the BBB, providing biochemical support to those cells.[7] The BBB is distinct from the quite similar blood–cerebrospinal fluid barrier, which is a function of the choroidal cells of the choroid plexus, and from the blood–retinal barrier, which can be considered a part of the whole realm of such barriers.[8]
Several areas of the human brain are not on the brain side of the BBB. Some examples of this include the circumventricular organs, the roof of the third and fourth ventricles, capillaries in the pineal gland on the roof of the diencephalon and the pineal gland. The pineal gland secretes the hormone melatonin "directly into the systemic circulation",[9] thus melatonin is not affected by the blood–brain barrier.[10]
Development
Originally, experiments in the 1920s seemed to show (wrongly) that the blood–brain barrier (BBB) was still immature in newborns. This was due to an error in methodology (the osmotic pressure was too high and the delicate embryonal capillary vessels were partially damaged). It was later shown in experiments with a reduced volume of the injected liquids that the markers under investigation could not pass the BBB. It was reported that those natural substances such as albumin, α-1-fetoprotein or transferrin with elevated plasma concentration in the newborn could not be detected outside of cells in the brain. The transporter P-glycoprotein exists already in the embryonal endothelium.[11]
The measurement of brain uptake of acetamide, antipyrine, benzyl alcohol, butanol, caffeine, cytosine, phenytoin, ethanol, ethylene glycol, heroin, mannitol, methanol, phenobarbital, propylene glycol, thiourea, and urea in ether-anesthetized newborn vs. adult rabbits shows that newborn rabbit and adult rabbit brain endothelia are functionally similar with respect to lipid-mediated permeability.[12] These data confirmed that no differences in permeability could be detected between newborn and adult BBB capillaries. No difference in brain uptake of glucose, amino acids, organic acids, purines, nucleosides, or choline was observed between adult and newborn rabbits.[12] These experiments indicate that the newborn BBB has restrictive properties similar to that of the adult. In contrast to suggestions of an immature barrier in young animals, these studies indicate that a sophisticated, selective BBB is operative at birth.
Function
The blood–brain barrier acts effectively to protect the brain from circulating pathogens. Accordingly, blood-borne infections of the brain are rare.[1] Infections of the brain that do occur are often difficult to treat. Antibodies are too large to cross the blood–brain barrier, and only certain antibiotics are able to pass.[13] In some cases, a drug has to be administered directly into the cerebrospinal fluid (CSF) where it can enter the brain by crossing the blood–cerebrospinal fluid barrier.[14][15]
The blood–brain barrier may become leaky in select neurological diseases, such as amyotrophic lateral sclerosis, epilepsy, brain trauma and edema, and in systemic diseases, such as liver failure.[1] The blood–brain barrier becomes more permeable during inflammation,[1] allowing antibiotics and phagocytes to move across the BBB. However, this also allows bacteria and viruses to infiltrate the blood–brain barrier.[13][16] Examples of pathogens that can traverse the blood–brain barrier include Toxoplasma gondii which causes toxoplasmosis, spirochetes like Borrelia (Lyme disease), Group B streptococci which causes meningitis in newborns,[17] and Treponema pallidum which causes syphilis. Some of these harmful bacteria gain access by releasing cytotoxins like pneumolysin[18] which have a direct toxic effect on brain microvascular endothelium[19] and tight junctions.
Circumventricular organs
Circumventricular organs (CVOs) are individual structures located adjacent to the fourth ventricle or third ventricle in the brain, and are characterized by dense capillary beds with permeable endothelial cells unlike those of the blood–brain barrier.[20][21] Included among CVOs having highly permeable capillaries are the area postrema, subfornical organ, vascular organ of the lamina terminalis, median eminence, pineal gland, and three lobes of the pituitary gland.[20][22]
Permeable capillaries of the sensory CVOs (area postrema, subfornical organ, vascular organ of the lamina terminalis) enable rapid detection of circulating signals in systemic blood, while those of the secretory CVOs (median eminence, pineal gland, pituitary lobes) facilitate transport of brain-derived signals into the circulating blood.[20][21] Consequently, the CVO permeable capillaries are the point of bidirectional blood–brain communication for neuroendocrine function.[20][22][23]
Specialized permeable zones
The border zones between brain tissue "behind" the blood–brain barrier and zones "open" to blood signals in certain CVOs contain specialized hybrid capillaries that are leakier than typical brain capillaries, but not as permeable as CVO capillaries. Such zones exist at the border of the area postrema–nucleus tractus solitarii (NTS),[24] and median eminence–hypothalamic arcuate nucleus.[23][25] These zones appear to function as rapid transit regions for brain structures involved in diverse neural circuits – like the NTS and arcuate nucleus – to rapidly control neurohumoral integration.[23][24] The permeable capillary zone shared between the median eminence and hypothalamic arcuate nucleus is augmented by wide pericapillary spaces, facilitating bidirectional flow of solutes between the two structures, and indicating that the median eminence is not only a secretory organ, but may also be a sensory CVO.[23][25]
Clinical significance
As a drug target
The blood–brain barrier (BBB) is formed by the brain capillary endothelium and excludes from the brain ∼100% of large-molecule neurotherapeutics and more than 98% of all small-molecule drugs.[1] Overcoming the difficulty of delivering therapeutic agents to specific regions of the brain presents a major challenge to treatment of most brain disorders. In its neuroprotective role, the blood–brain barrier functions to hinder the delivery of many potentially important diagnostic and therapeutic agents to the brain. Therapeutic molecules and antibodies that might otherwise be effective in diagnosis and therapy do not cross the BBB in adequate amounts.
Mechanisms for drug targeting in the brain involve going either "through" or "behind" the BBB. Modalities for drug delivery to the brain in unit doses through the BBB entail its disruption by osmotic means, or biochemically by the use of vasoactive substances such as bradykinin, [26] or even by localized exposure to high-intensity focused ultrasound (HIFU).[27] Other methods used to get through the BBB may entail the use of endogenous transport systems, including carrier-mediated transporters such as glucose and amino acid carriers, receptor-mediated transcytosis for insulin or transferrin, and the blocking of active efflux transporters such as p-glycoprotein. However, vectors targeting BBB transporters, such as the transferrin receptor, have been found to remain entrapped in brain endothelial cells of capillaries, instead of being ferried across the BBB into the targeted area.[28][29]Methods for drug delivery behind the BBB include intracerebral implantation (such as with needles) and convection-enhanced distribution. Mannitol can be used in bypassing the BBB.
Nanoparticles
Nanotechnology may also help in the transfer of drugs across the BBB.[30][31] Recently, researchers have been trying to build liposomes loaded with nanoparticles to gain access through the BBB. More research is needed to determine which strategies will be most effective and how they can be improved for patients with brain tumors. The potential for using BBB opening to target specific agents to brain tumors has just begun to be explored.
Delivering drugs across the blood–brain barrier is one of the most promising applications of nanotechnology in clinical neuroscience. Nanoparticles could potentially carry out multiple tasks in a predefined sequence, which is very important in the delivery of drugs across the blood–brain barrier.
A significant amount of research in this area has been spent exploring methods of nanoparticle-mediated delivery of antineoplastic drugs to tumors in the central nervous system. For example, radiolabeled polyethylene glycol coated hexadecylcyanoacrylate nanospheres targeted and accumulated in a rat gliosarcoma.[32] However, this method is not yet ready for clinical trials, due to the accumulation of the nanospheres in surrounding healthy tissue. Another, more promising approach, is the coating of polyalkylcyanoacrylate or poly-lactic-co-glycolic acid (PLGA) nanoparticles with polysorbate 80 or poloxamer 188. Due to this coating the particles adsorb apolipoproteins E or A-1 from the blood and thus interact with the signalling protein LRP1 or with the scavenger receptor followed by transcytosis across the blood–brain barrier into the brain.[33] These particles loaded with doxorubicin for the treatment of glioblastomas are presently in Clinical Phase I. Recently, a novel class of multifunctional nanoparticles known as magneto-electric nanoparticles (MENs) has been discovered for externally controlled targeted delivery and release of drug(s) across BBB as well as wireless stimulation of cells deep in the brain. This approach depends more on the field control and less on the cellular microenvironment. In vitro and in vivo (on mice) experiments to prove the feasibility of using MENs to release a drug across BBB on demand and wirelessly stimulate the brain have been conducted by the research group of Prof. Sakhrat Khizroev at Florida International University (FIU).[34]
Vascular endothelial cells and associated pericytes are often abnormal in tumors and the blood–brain barrier may not always be intact in brain tumors. Also, the basement membrane is sometimes incomplete. Other factors, such as astrocytes, may contribute to the resistance of brain tumors to therapy.[35][36]
Peptides
Peptides are able to cross the blood–brain barrier (BBB) through various mechanisms, opening new diagnostic and therapeutic avenues.[37] However, their BBB transport data are scattered in the literature over different disciplines, using different methodologies reporting different influx or efflux aspects. Therefore, a comprehensive BBB peptide database (Brainpeps) was constructed to collect the BBB data available in the literature. Brainpeps currently contains BBB transport information with positive as well as negative results. The database is a useful tool to prioritize peptide choices for evaluating different BBB responses or studying quantitative structure-property (BBB behaviour) relationships of peptides. Because a multitude of methods have been used to assess the BBB behaviour of compounds, these methods and their responses are classified. Moreover, the relationships between the different BBB transport methods have been clarified and visualized.[38]
Casomorphin is a heptapeptide and could be able to pass the BBB.[39]
Disease
Meningitis
Meningitis is an inflammation of the membranes that surround the brain and spinal cord (these membranes are known as meninges). Meningitis is most commonly caused by infections with various pathogens, examples of which are Streptococcus pneumoniae and Haemophilus influenzae. When the meninges are inflamed, the blood–brain barrier may be disrupted.[13] This disruption may increase the penetration of various substances (including either toxins or antibiotics) into the brain. Antibiotics used to treat meningitis may aggravate the inflammatory response of the central nervous system by releasing neurotoxins from the cell walls of bacteria - like lipopolysaccharide (LPS).[40] Depending on the causative pathogen, whether it is bacterial, fungal, or protozoan, treatment with third-generation or fourth-generation cephalosporin or amphotericin B is usually prescribed.[41]
Brain abscess
A brain or cerebral abscess, like other abscesses, is caused by inflammation and collection of lymphatic cells and infected material originating from a local or remote infection. A brain abscess is a rare, life-threatening condition. Local sources may include infections of the ear, the oral cavity and teeth, the paranasal sinuses, or an epidural abscess. Remote sources may include infections in the lung, heart or kidney. A brain abscess may also be caused by head trauma or as a complication of surgery. In children cerebral abscesses are usually linked to congenital heart disease.[42] In most cases, 8–12 weeks of antibacterial therapy is required.[13]
Epilepsy
Epilepsy is a common neurological disease that is characterized by recurrent and sometimes untreatable seizures. Several clinical and experimental data have implicated the failure of blood–brain barrier function in triggering chronic or acute seizures.[43][44][45][46][47] Some studies implicate the interactions between a common blood protein (albumin) and astrocytes.[48] These findings suggest that acute seizures are a predictable consequence of disruption of the BBB by either artificial or inflammatory mechanisms. In addition, expression of drug resistance molecules and transporters at the BBB are a significant mechanism of resistance to commonly used anti-epileptic drugs.[49][50]
Multiple sclerosis
Multiple sclerosis (MS) is considered to be an auto-immune and neurodegenerative disorder in which the immune system attacks the myelin that protects and electrically insulates the neurons of the central and peripheral nervous systems. Normally, a person's nervous system would be inaccessible to the white blood cells due to the blood–brain barrier. However, magnetic resonance imaging has shown that when a person is undergoing an MS "attack," the blood–brain barrier has broken down in a section of the brain or spinal cord, allowing white blood cells called T lymphocytes to cross over and attack the myelin. It has sometimes been suggested that, rather than being a disease of the immune system, MS is a disease of the blood–brain barrier.[[51]](https://portal.issn.org/resource/ISSN/"Biomarkers indicative of blood–brain barrier disruption in multiple sclerosis") The weakening of the blood–brain barrier may be a result of a disturbance in the endothelial cells on the inside of the blood vessel, due to which the production of the protein P-glycoprotein is not working well.[52]
There are currently active investigations into treatments for a compromised blood–brain barrier. It is believed that oxidative stress plays an important role into the breakdown of the barrier. Anti-oxidants such as lipoic acid may be able to stabilize a weakening blood–brain barrier.[53]
Neuromyelitis optica
Neuromyelitis optica, also known as Devic's disease, is similar to and is often confused with multiple sclerosis. Among other differences from MS, a different target of the autoimmune response has been identified. Patients with neuromyelitis optica have high levels of antibodies against a protein called aquaporin 4 (a component of the astrocytic foot processes in the blood–brain barrier).[54]
De Vivo disease
De Vivo disease (also known as GLUT1 deficiency syndrome) is a rare condition caused by inadequate transportation of the sugar glucose across the blood–brain barrier, resulting in developmental delays and other neurological problems. Genetic defects in glucose transporter type 1 (GLUT1) appears to be the primary cause of De Vivo disease.[55][56]
Alzheimer's disease
Some evidence indicates that disruption of the blood–brain barrier in Alzheimer's disease allows blood plasma containing amyloid beta (Aβ) to enter the brain where the Aβ adheres preferentially to the surface of astrocytes.[57] These findings have led to the hypotheses that (1) breakdown of the blood–brain barrier allows access of neuron-binding autoantibodies and soluble exogenous Aβ42 to brain neurons and (2) binding of these autoantibodies to neurons triggers and/or facilitates the internalization and accumulation of cell surface-bound Aβ42 in vulnerable neurons through their natural tendency to clear surface-bound autoantibodies via endocytosis. Eventually the astrocyte is overwhelmed, dies, ruptures, and disintegrates, leaving behind the insoluble Aβ42 plaque. Alzheimer's disease may be caused (or more likely, aggravated) by a breakdown in the blood–brain barrier.[58]
HIV encephalitis
A 2002 review indicated that latent HIV can cross the blood–brain barrier inside circulating monocytes in the bloodstream ("Trojan horse theory") within the first 14 days of infection.[59] Once inside, these monocytes become activated and are transformed into macrophages. Activated macrophages release virions into the brain tissue proximate to brain microvessels. These viral particles likely attract the attention of sentinel brain microglia and perivascular macrophages initiating an inflammatory cascade that may cause a series of intracellular signaling in brain microvascular endothelial cells and damage the functional and structural integrity of the BBB.[60]
Systemic inflammation
During systemic inflammation, whether in the form of infection or sterile inflammation, the BBB may become leaky to blood-borne compounds.[61] Such changes may be part of a productive host response to systemic inflammation, or may lead to deleterious consequences within the central nervous system. These changes may also affect the course of multiple sclerosis or Alzheimer's disease.[62][63]
History
Paul Ehrlich was a bacteriologist studying staining, a procedure that is used in many microscopy studies to make fine biological structures visible using chemical dyes.[64] As Ehrlich injected some of these dyes (notably the aniline dyes that were then widely used), the dye stained all of the organs of some kinds of animals except for their brains.[64] At that time, Ehrlich attributed this lack of staining to the brain simply not picking up as much of the dye.[65]
However, in a later experiment in 1913, Edwin Goldmann (one of Ehrlich's students) injected the dye directly into the cerebrospinal fluids of animal brains. He found then the brains did become dyed, but the rest of the body did not, demonstrating the existence of a compartmentalization between the two. At that time, it was thought that the blood vessels themselves were responsible for the barrier, since no obvious membrane could be found. The concept of the blood–brain barrier (then termed hematoencephalic barrier) was proposed by a Berlin physician, Lewandowsky, in 1900.[66]
See also
Blood–air barrier – Membrane separating alveolar air from blood in lung capillaries
Blood–ocular barrier – A physical barrier between the local blood vessels and most parts of the eye itself
Blood–retinal barrier – Part of the blood–ocular barrier that prevents certain substances from entering the retina
Blood–testis barrier – A physical barrier between the blood vessels and the seminiferous tubules of the animal testes
Blood–thymus barrier – A barrier formed by the continuous blood capillaries in the thymic cortex
Choroid plexus for blood–cerebrospinal fluid barrier
Ventricular system
Capillary
Mast cell
Mobile phone radiation and health
Endothelium
Glymphatic system
Lina Stern – Soviet biochemist